The mind, the world, and the cookie cutter paradigm
Particles and brains — the instruments of manifestation
In my last mailing, I quoted Sri Aurobindo’s characterization of our own kind of consciousness:
Mind in its essence is a consciousness which measures, limits, cuts out forms of things from the indivisible whole and contains them as if each were a separate integer. Even with what exists only as obvious parts and fractions, Mind establishes this fiction of its ordinary commerce that they are things with which it can deal separately and not merely as aspects of a whole. For, even when it knows that they are not things in themselves, it is obliged to deal with them as if they were things in themselves; otherwise it could not subject them to its own characteristic activity. It is this essential characteristic of Mind which conditions the workings of all its operative powers, whether conception, perception, sensation or the dealings of creative thought. It conceives, perceives, senses things as if rigidly cut out from a background or a mass and employs them as fixed units of the material given to it for creation or possession. All its action and enjoyment deal thus with wholes that form part of a greater whole, and these subordinate wholes again are broken up into parts which are also treated as wholes for the particular purposes they serve. Mind may divide, multiply, add, subtract, but it cannot get beyond the limits of this mathematics....
The sentence emphasized here (by me) expresses an idea I have dubbed the “cookie cutter paradigm” (CCP). It is the idea that the synchronic multiplicity of the world — its multiplicity at any one time — rests on surfaces that carve up space much as cookie cutters carve up rolled-out pastry.
I suppose the CCP will seem rather self-evident to anyone uninitiated into the mysteries of the quantum world. After all, every thing in visual experience has spatial extent and is therefore, at least conceptually, divisible by cutting. The literal meaning of the Greek word ἄτομος is “uncuttable.” The reason it is usually translated as “indivisible” is that, until quantum mechanics came along, the parts of material objects were defined by boundaries, and ultimate material parts were considered indivisible either because the stuff enclosed by their boundaries was for some reason incapable of being cut further or because they were pointlike entities.
The CCP appears to be hardwired: the way in which the mammalian brain processes visual information seems to guarantee that the result — the visual aspect of the phenomenal world — is a world of objects whose shapes are bounding surfaces. In the next few paragraphs I shall present in outline what the neuroscientific study of perception has brought to light about the construction (by the brain or the mind) of the visual world.1
The neural analysis of the visual field — the optical images falling on the retinas in our eyes — capitalizes on contrast information. Data arriving from homogeneously colored and evenly lit regions of the visual field are largely ignored. The corresponding regions of the visual world are filled in — much like children’s coloring books — on the basis of changes in color or brightness across boundaries in the visual field. For a demonstration, refer to this (or some other) demonstration of the retina’s blind spot. Apart from being remarkably economical with respect to the amount of sensory data needed to construct the visual world, this filling mechanism appears to play a significant role in achieving the constancy of color perception (i.e., our ability to perceive fairly constant surface colors despite changes in illumination). The checker shadow illusion illustrated below may serve as an example: square A looks darker than square B even though they are both exactly the same shade of gray.
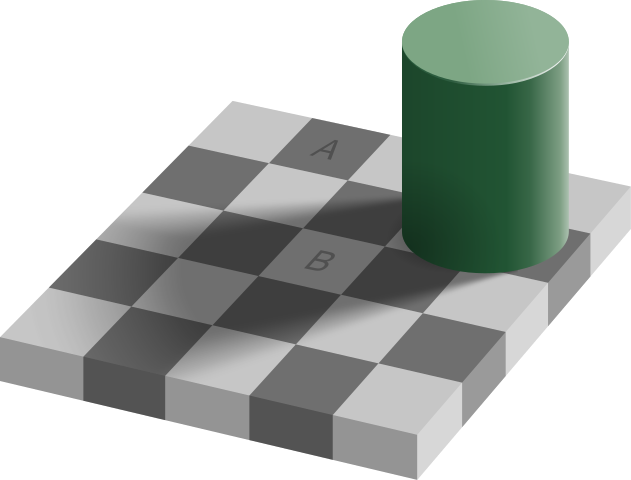
The extraction of discontinuities from the visual field already begins in the retinas, which can be described as rather sophisticated computing devices in their own right.2 Situated behind the retina’s receptor cells — the light-sensitive rods and cones — there are several layers of ganglion cells, each sending information about a particular aspect of the visual field to the visual cortex (the part of the cerebral cortex that is responsible for processing visual information).
The receptive field of a ganglion cell — the part of the retina from which it receives input — is circular. The receptive fields of about 90% of these cells are divided into a small central region and a larger surrounding region. Some of these cells (called “on-center” cells) are excited (their firing rate is increased) by illumination of the center and inhibited (their firing rate is decreased) by illumination of the surround. Others (the “off-center” cells) are inhibited from the center and excited from the surround. An on-center cell responds best to a circular spot of light of a specific size, responds well to a bright line that just covers the center, and does not respond if both center and surround are fully and equally illuminated.
From the retinas, visual information flows via two nests of cells deep in the brain (the lateral geniculate bodies) to the visual cortex at the back of the brain. Whereas many geniculate cells respond to diffuse light, even if weakly, cortical cells are more choosy. When, in 1958, David Hubel and Torsten Wiesel made one of the first technically successful recordings from an individual neuron in the cortex of a cat, they “tried everything short of standing on our heads to get it to fire”.3 That neuron turned out to be a complex cell, which is the commonest type of neuron in the primary visual cortex (a.k.a. “V1”). (The rest have center-surround receptive fields and are color selective.) The most effective way to elicit a response from a complex cell is to sweep a line across its receptive field, in a direction perpendicular to the line’s orientation — a bright line on a dark background, a dark line on a bright background, or the boundary between areas of different brightness.
In V1, ten to twenty percent of the orientation selective cells have another specific feature: they are end-stopped. (Further upstream, in area V2, at least half of the orientation selective cells are end-stopped.) An ordinary complex cell usually shows length summation: the longer the stimulus line, the stronger the response, until the line is as long as the cell’s receptive field. For an end-stopped cell, lengthening the line improves the response up to some limit, but exceeding that limit in one or both directions results in a weaker response. The optimal stimulus for an end-stopped cell is a line that extends for a certain distance and no further. For a cell that responds to edges and is end stopped at one end only, a corner is ideal. For a cell that responds to bright or dark lines and is stopped at both ends, the optimum stimulus is a short line or a line that curves so that it is has the right orientation in the activating region of the cell and the wrong orientation — different by 20 degrees or more — in the flanking regions. We can thus view end-stopped cells as sensitive to corners, to curvature, or to sudden breaks in lines.
From area V1, visual information is broadcast to a number of regions, which can be grouped into two general streams. The dorsal stream (a.k.a. the “where stream”) contains topographic maps of the visual field in which individual neurons are sensitive to information about movement and spatial location. The ventral stream (a.k.a. the “what stream”) contains topographic maps that are sensitive to shape, color, and surface texture.4 (A topographic map is a layer of the cerebral cortex in which cells map a particular phenomenal variable in such a way that adjacent cells generally correspond to adjacent locations in the visual field.) Ascending the visual processing hierarchy, two trends are observed. Neurons respond to their preferred stimulus over progressively larger regions of the visual field, and they get progressively more specific in regard to the visual attributes to which they respond: neurons further up the ventral stream respond most vigorously to particular objects such as faces, buildings, or cars.5
The long and the short of it is that the construction of the visual world appears to begin with the extraction of line segments from the visual field, and to proceed by a progressive synthesis. First line segments are integrated into contours, then contours are integrated into something analogous to the wire frames of computer-aided design software, and finally the “wire frames” are covered with colored and textured surfaces. (It also involves the detection and analysis of motion and, as just mentioned, proceeds further to an increasing specificity of object recognition.)
In the neuroscience of perception, it has often been assumed that vision in humans has a single function: to provide a unified internal representation of the external world. Given this generally implicit and unquestioned assumption,6 the construction of the phenomenal world (by the mind and/or the brain) is seen as the re-construction of a mind-independently existing world. To recognize the flaw in this assumption, let us once more invoke our imaginary neuroscientist Alice. Having placed something red and squarish in Bob’s visual field, she observes that a certain set of neurons in Bob’s visual cortex respond. Then, placing something green and roundish in his visual field, she finds that a different set of neurons respond. In this way she establishes a correspondence between what she perceives as located in the place Bob is looking at and what she observes in Bob’s visual cortex. This correspondence therefore is not between a self-existent object in front of Bob and neural firings in Bob’s brain. Insofar as something in Bob’s visual world is a re-presentation, it is a re-presentation of something that has previously occurred in his visual world, not a re-presentation of something in a mind-independently existing world.
A related idea (previously mentioned here) is that neural representations are encodings. The information our brains extract from the images falling on our retinas is said to be encoded in patterns of electrochemical pulses. If these patterns are to be experienced as (or to give rise to experiences of) a world extended in space and time, they have to be decoded. They have to be interpreted by something or someone acquainted with the expanse of space and the passing of time, and (as has been argued in the aforementioned post) this acquaintance is not something that neural representations can provide.
Let’s draw some conclusions. The sensory data our brains are believed to receive are inaccessible to us. What is empirically accessible is objects appearing to exist outside us, including the neural firing patterns observed by Alice and the correlations that exist between them and other objects perceived by her. Science — actual science, not metaphysics masquerading as science — has access to the visual world as well as to neural processes involved in the construction of the visual world, insofar as they themselves belong to the visual world. Lines, contours and such are not extracted from independent objects. We only know them as elements from which the visual worlds of both Alice and Bob are constructed.
Doesn’t this remind you of something? Remember the distinction quantum mechanics requires us to draw between a “classical domain” and a “quantum domain.” The former is accessible to sensory experience and therefore capable of being described in terms of concepts that owe their meanings in part to the spatiotemporal structure of human sensory experience. The latter is not accessible to human sensory experience and therefore cannot be expected to be describable in these terms. To Niels Bohr, this meant that the physical content of quantum mechanics was “exhausted by its power to formulate statistical laws governing observations obtained under conditions specified in plain language”.7 In the narrower sense of “physical content,” he is right up to the present day. There are, however, certain ontological conclusions that can be drawn from the quantum-mechanical correlations between classically describable events, and one of them is that the difference between the two domains is the difference between the manifested world and its manifestation.
Quantum mechanics allows us to describe the manifestation of the world in these simple terms: By entering into reflexive spatial relations, a single Ultimate Constituent gives rise to (i) what looks like a multiplicity of relata if the reflexive quality of the relations is ignored, and (ii) what looks like a substantial expanse if the spatial quality of the relations is reified. In other words: By entering into reflexive relations, this Ultimate Constituent engenders both matter and space, for space may be defined as the totality of existing spatial relations, while matter may be defined as the corresponding (apparent) multitude of relata.
This transition from the unity of the Ultimate Constituent to the multiplicity of the manifested world is progressive. Subatomic particles, non-visualizable atoms, and partly visualizable molecules mark the stages of this progression. Instead of being constituent parts of the manifested world, they are instrumental in its manifestation.
Across the stages of that transition, the differentiatedness of the manifested world — its being divided into distinguishable regions of space, distinguishable objects, and distinguishable properties — is gradually realized. But how can we describe the intermediate stages, at which the distinguishability of regions, of objects, and of properties is as yet incompletely realized? The answer is that whatever is not inherently distinct can only be described in terms of probability distributions over events that are inherently distinct, namely, the possible outcomes of measurements. What is instrumental in the manifestation of the world can only be described in terms of correlations between events that happen (or could happen) in the manifested world. Incidentally, as has already been observed, this goes a long way towards explaining why the general theoretical framework of contemporary physics is a probability calculus, and why the events to which it serves to assign probabilities are measurement outcomes.
Before proceeding, it will be useful to remember that, when talking about quantum objects, a clear distinction between object types and individual objects must be maintained. As we have seen here, there is no single, unified way of talking about quantum objects. There is the axiomatic way, which deals with particle types, their interactions and possible interconversions, and their composite or bound states, including nuclei, atoms, and molecules. There is the operational way, which comprises classical and quasi-classical methods of producing and analyzing statistical data. And there is the contextual way, which refers to individual quantum objects.
Now, do you see pattern? Or at least an analogy? Atoms and subatomic particles are instrumental in the manifestation of the world. Their contextuality does not allow us to think of them as constituent parts of the world. Our entire theoretical and experimental knowledge of them — whether as individuals, as statistical ensembles, or as types (or Lockean substances or natural kinds) — derives from correlations between events in the manifested world. Similarly, patterns of electrochemical pulses in brains are instrumental in manifesting the world to us, in our experience. Our knowledge of their instrumentality in the construction of the visual world is based on correlations between objects or events within the visual world, such as firing patterns in Bob’s visual cortex and objects in Bob’s visual field, both of which are known to Alice (our generic neuroscientist) only because they exist or occur in her visual world.
This leads to the following conclusions. There is no such thing as the manifested world. The world is manifested to an evolving consciousness, and as consciousness is evolving so is the manifested world — and this not because we get more efficient at messing with the world but because the world is what it is only because it is experienced the way it is experienced. Thus both particles and brains are instrumental in the evolution of the manifested world, or in the evolutionary manifestation of the world.
Evolution, as previously stated, is far from finished. We speak of the evolution of life, the evolution of mind, the eventual evolution of supermind. But what do these terms really mean? When life emerged, what essentially emerged was the power to execute ideas, to realize them in action and form. When mind emerged, what essentially emerged was the power to generate ideas. The true nature of these principles is obscured by the Houdiniesque nature of this evolutionary manifestation, due to which the emergence of life required the creation of increasingly complex organisms, and the emergence of mind required the creation of increasingly complex nervous systems. When supermind will emerge, what will emerge is essentially the power to develop into expressive ideas the infinite Quality/Delight at the heart of reality. Then the entire creative process — the development of Quality into Form using mind to generate expressive ideas and the formative force of life to execute them — will be conscious and deliberate, and our anatomical insides and their physiology will no longer be needed. They can be discarded much like a scaffolding or the chrysalis of a butterfly.
There remains one loose end to be tied. Above I wrote that “whatever is not inherently distinct can only be described in terms of probability distributions over events that are inherently distinct.” To what do the possible outcomes of a measurement owe their inherent distinctness? Where exactly does it inhere? Because of the manner in which the world is manifested to us, the shapes of things are bounding surfaces, and the parts of things — to the extent they can be visually resolved — conform to the CCP. But it is an essential feature of the outcome-indicating properties of a measurement apparatus — the respective “pointer positions” by which the possible outcomes are indicated — can be visually resolved. And so it is to the CCP (a characteristic of mental consciousness and of the world as manifested to us) that the possible outcomes of a measurement owe their inherent distinctness. The distinctness inheres in the consciousness for which the world exists. It doesn’t belong to a reality existing out of relation to consciousness.
D.H. Hubel, Eye, Brain, and Vision (Scientific American Library, 1995); J.T. Enns, The Thinking Eye, the Seeing Brain (Norton, 2004); M. Velmans, Understanding Consciousness (2nd edition, Routledge, 2009).
B. Roska and F. Werblin, Vertical interactions across ten parallel, stacked representations in the mammalian retina, Nature 410, 538–587 (2001); F. Werblin and B. Roska, The Movies in Our Eyes, Scientific American (April 2007), pp. 73–79.
Hubel, op. cit.
A. David Milner and Melvyn A. Goodale (The Visual Brain in Action, Oxford University Press, 2006) have challenged the notion that the two streams merely handle different aspects of visual perception. Instead, they propose that the anatomical distinction between the ventral and dorsal streams corresponds to the distinction between perceptual representation and visual motor control.
Enns, op. cit., p. 53.
Beginning with Darwin’s “horrid doubt ... whether the convictions of man’s mind, which has been developed from the mind of the lower animals, are of any value or at all trustworthy,” this assumption has been questioned by evolutionary theorists. Steven Pinker opines that “[o]ur minds evolved by natural selection to solve problems that were life-and-death matters to our ancestors, not to commune with correctness,” and Patricia Churchland argues that “a fancier style of representing is advantageous so long as it is geared to the organism’s way of life and enhances the organism’s chances of survival. Truth, whatever that is, definitely takes the hindmost.” (F. Darwin, The Life and Letters of Charles Darwin, Vol. 1, pp. 315–316, John Murray, 1887; S. Pinker, How the Mind Works, p. 561, Norton, 1997; P. Churchland, Epistemology in the Age of Neuroscience, Journal of Philosophy 84, 544–553, 1987.)
N. Bohr, Collected Works, Vol. 10, p. 159 (Elsevier, 1999).